Study
Objectives
· To define or describe concepts such as
catecholamines, nanism, pituitary dwarfism, pseudo-hypo-para-thyroidism,
somatomedins, somatostatin, and somatotropin.
· To describe the regulation of extracellular and intracellular Ca2+, the bone
structure, remodelling and the function of osteoblasts, osteoclasts and
osteocytes. To describe the biosynthesis of calcitonin, parathyroid hormone,
vitamin D, mineralocorticoids, glucocorticoids, adrenaline and noradrenaline.
To describe osteoporosis, osteomalacia, and rachitis. To describe the Ca2+ and phosphate balance in healthy persons.
· To explain the effects of calcitonin, parathyroid hormone, vitamin D, mineralocorticoids,
glucocorticoids, androgens and oestrogens from the adrenal cortex, and
adrenaline/noradrenaline. To explain phenomena such as phaeochromocytoma,
shock, adrenogenital syndrome, virilization and pubertas praecox.
· To use the above concepts in problem solving and case histories.
Principles
· The
sympatho-adrenergic system give rise to the fright, flight or fight reactions
that are all acute stress situations.
· The
adrenal cortex is concerned with the carbohydrate metabolism and with the
electrolyte balance.
· The
parathyroid hormone and vitamin D maintain normal levels of calcium and
phosphate in the body.
Definitions
· Calcium
concentration (total) in plasma: 2.2-2.7 mM.
Half of the total calcium is ionized.
· Catecholamines are substances consisting of catechol (an
aromatic structure with two hydroxyl groups) linked to an amine. The important
catecholamines in humans are adrenaline, noradrenaline and dopamine.
· Cushing’s
disease is hypercorticism (increased
glucocorticoid production) caused by a pituitary basophilic adenoma.
· Cushing’s
syndrome refers to the consequences of increased
plasma glucocorticoid concentration from any source.
· Growth
hormone releasing hormone (GHRH) is a peptide
produced by GHRH cells in the hypothalamus. GHRH stimulates the release of
growth hormone (Somatotropin) from the adenohypophysis.
· Hypercalcaemia is an increase in the plasma concentration of ionized calcium. The condition
is characterised by neurological signs (hyporeflexia, lethargy or coma). When
Ca2+ is deposited in the inner medulla of the kidney, ADH
resistance develops with polyuria and polydipsia.
· Hypocalcaemia is a decrease in the plasma concentration of ionized Ca2+ (cramps,
tetany, twitching and tingling of the fingers). Hypocalcaemia is caused by
renal failure or by thyroidectomy and parathyroidectomy.
· Hyperparathyroidism. Primary hyperparathyroidism is caused by
parathyroid adenomas or by hyperplasia. Secondary hyperparathyroidism is
compensatory hypertrophia due to hypocalcaemia.
· Hypoparathyroidism. The idiopathic form is a rare autoimmune destruction of the parathyroid (with
hypocalcaemia).
· Infantile
nanism is a term used to indicate that the
somatic age of a child is below its chronological age.
· Nanism or dwarf
growth expresses that the
height of an adult is below a certain limit. In the Anglo-Saxon countries the
limit is 1.40 m for females and 1.50 m for males.
· Pituitary
dwarfism refers to low pituitary secretion of GH
or insensitive target organ receptors (African pygmies).
· Pseudo-hypo-para-thyroidism is used for conditions, where target-organs (bone, kidneys, and gut) are resistant
to PTH. The plasma- [Ca2+ ] is low, plasma-[phosphate] is high, and basic phosphatase activity is high.
· Somatomedins are small peptides (5 kDa) produced in the liver, promote bone growth and
protein synthesis. Somatomedin C is also called insulin-like growth-factor -1
(IGF-1). The hepatic production of somatomedins is stimulated by growth
hormone. Somatomedins stimulate the hypothalamic secretion of somatostatin.
· Somatotropin
or growth hormone (GH) from the anterior
pituitary stimulates body growth in humans. GHRH stimulates and somatostatin
inhibits the release of GH. Growth hormone stimulates the hepatic production
of Somatomedin C (IGF-1).
· Somatostatin or growth hormone inhibiting hormone (GHIH) is a cyclic peptide, which is
produced in the pancreatic islets and in the CNS, where it is co-transmitter
with noradrenaline. GHIH inhibits the release of growth hormone from the
adenohypophysis and is therefore also called somatotropin releasing inhibiting
factor, SRIF.
Essentials
This
paragraph deals with 1. Growth hormone,
2. Parathyroid hormone. Calcium and
phosphate homeostasis, 3. Adrenal corticoids,
4. Adrenal catecholamines, and 5. Vitamin
D and bone minerals.
1.
Growth hormone (GH)
Growth
hormone is
the main stimulator of body growth in humans. Growth hormone is produced in
the pituitary, stimulated by hypothalamic growth hormone releasing hormone
(GHRH) and inhibited by
hypothalamic somatostatin (Fig.
30-1). Pituitary growth hormone stimulates the hepatic production of
transcription factors such as Somatomedin C (IGF-1) and its specific IGF-binding
protein 1 (IGF-BP1). Actually,
it is somatomedin C that stimulates body growth - both bone and muscle growth.
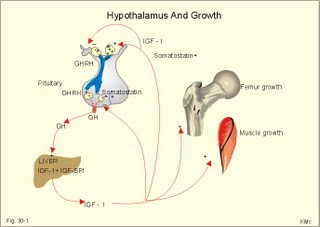
Fig.
30-1: Pituitary GH and hepatic IGF-1 secretion in the
hypothalamic-pituitary feedback system.
Growth hormone releasing hormone (GHRH) is
produced by GHRH cells in the hypothalamus and reaches the adenohypophysis via
the portal system. GHRH stimulates the release of growth hormone (GH) from the
adenohypophysis (Fig. 30-1).
Growth hormone and insulin are important
anabolic hormones in humans, although growth hormone has many
insulin-antagonistic effects. Growth hormone is released as hunger
spikes during the day and during sleep.
Growth
hormone (GH) produced in the placenta differs
from the pituitary GH by a few Amino acid residues. Placental
GH suppresses release of maternal, pituitary GH during Pregnancy.
Placental GH stimulates maternal metabolism and foetal cell proliferation and
hypertrophia.
Foetal
thyroid hormones stimulate brain development,
and foetal insulin stimulates foetal
growth, cellular glucose uptake and glucose utilisation. Paracrine and
autocrine growth Factors
are also important for foetal growth: Insulin-like growth factor-II (IGF-II),
nerve Growth factors (NGF), epidermal growth factor (EGF), and platelet
derived growth factor (PDGF).
The pituitary
GH has an endocrine effect on the production of the growth factor Somatomedin
C (IGF-1) and its specific binding protein in the liver. Hepatic IGF-1
circulates in plasma bound to IGF-binding
protein 1 (IGF-BP1). The binding
proteins are important, not only as a vehicle in plasma, but also for the
final binding of the hormone to its cell membrane receptor. One GH binds to
two cell receptor molecules, the intercellular portion of which activate
thyrosine kinasis. The kinasis phosphorylate the transcription factors that
modulate gene expression.
Hepatic
IGF-I stimulates bone formation, and GH stimulates the precondrocytes
directly. Locally released IGF-I stimulates
the condrocytes in healthy states, but it also has a regenerative function in
damaged tissues.
Pituitary GH also stimulates the production
of receptors for other growth factors. GH serves to commit a precursor cell to
a specific pathway, and IGF-I enhances its growth and replication.
Somatomedins inhibit the secretion of GH from the somatotropic cells of the
adenohypophysis. Somatomedins also stimulate the hypothalamic secretion of somatostatin (GHIH or somatotropin releasing inhibiting factor,
SRIF).
Tissue specific growth factors - just like
those important for foetal growth - are produced in damaged tissues, where
they are important for regeneration. Nervous tissues produce NGF, epidermal
tissues produce EGF, thrombocytes produce PDGF, and fibroblasts produce both fibroblast
growth factor (FGF) and transforming
growth factors (TGF-a,
and TGF-b).
Hepatocyte proliferation after liver damage is induced by both hepatocyte growth factor (HGF) and by hepatocyte stimulating substance (HSS). Growth factors are used in
regenerative therapy.
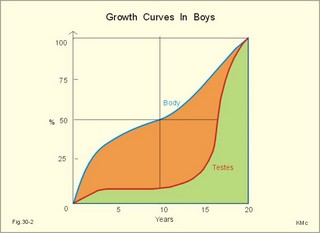
Fig.
30-2: 68-1: Growth curves in
boys - a similar pattern is found in girls.
The relative large growth of the testes at
puberty shows the importance of sex
hormones for early puberty growth (Fig. 30-2). In general, the growth
spurt in early puberty is caused by increased production of sex steroids (stimulated by luteinizing hormone and by follicle
stimulating hormone, FSH), because the sex steroids stimulate hypothalamus to
release more growth hormone.
Growth hormone and insulin are probably the
most important anabolic hormones in
the body - more effective than oestradiol and testosterone; however, GH has
many insulin-antagonistic effects. Human GH stimulates protein synthesis,
mitosis in cells, chondrogenesis, ossification, and phosphate balance
(increased renal phosphate reabsorption), while
increasing glycolysis (ie, anaerobic breakdown of glycogen). GH stimulates
hepatic glucose production (glycogenolysis) but not its gluconeogenesis. GH
enhances RNA synthesis, accelerates glucose uptake and antagonise the
lipolytic effect of adrenaline. - GH is produced in spikes during the day
(hunger spikes) and during deep sleep (EEG stage III and IV), and the
half-life of GH is 20 min.
Children with pan-hypopituitarism become infantile
dwarfs and later turn into juvenile
dwarfs, as they do not develop sexually.
Children with hyperpituitarism become giantesses or giants. The clinical diagnosis
is gigantismus.
Hypothyroid children (cretins) also become
dwarfs. The thyroid hormones are
necessary or permissive for normal
growth and development. Cretins are mentally
retarded, hypothyroid dwarfs.
Sex
steroids in high concentrations close the
epiphyseal lines. If this occurs early in life, the child also becomes an
infantile dwarf, but such an individual is often sexually active. The clinical
diagnosis is precocious puberty or pubertas
praecox.
Without proper insulin treatment, children with diabetes (mellitus) become dwarfs
(diabetic, infantile nanismus), because of the intracellular hypoglycaemia.
Poorly regulated diabetics also develop osteopenia (bone decalcification).
Genetic
factors are essential for optimal growth and
development, as shown by the strong correlation between the height of the
parents and the final height of the child. Tall people are high before the
puberty growth spurt, which is more or less the same for tall and shorter
people. - Persons with only one sex chromosome (X, 0) show retarded growth
from birth, whereas persons with an extra sex chromosome (XXY; XYY) become
tall.
Optimal growth depends upon optimal
nutrition (essential amino acids, vitamins, minerals, and fatty acids) and
optimal Neuroendocrine, metabolic control.
Optimal growth also
depends upon an optimal health. Most
disease states and all immuno-defence threatening treatments retard growth or
imply weight loss. Such disorders cause catabolic hormones to dominate and
induce anorexia.
2.
Parathyroid hormone (PTH). Calcium and phosphate homeostasis.
We
overlooked the existence of the parathyroid glands until the consequence of
their surgical removal was realised. Without the parathyroid, a person
develops tetanic cramps due to a
fall in the concentration of ionised calcium ([Ca2+]) in the blood
plasma (explained in Chapter 17). -
Already in 1909, MacCallum treated this condition successfully with calcium
salts.
The chief cells (C cells) of the parathyroid glands produce pre-proparathyroid hormone, which is cleaved in their endoplasmic
reticulum to proparathyroid hormone.
This in turn is cleaved in the Golgi apparatus to parathyroid hormone (PTH).
PTH is a single chain peptide with a molecular weight of 9500 Da.
PTH is water-soluble and binds to membrane
receptors on the surface of the target cells. Thus the PTH action is dependent
on second messengers. Both intracellular Ca2+ and cyclic adenosine
monophosphate (cAMP) are used.
Humans carry a single PTH gene on chromosome
11. The chief cells also produce a parathyroid
secretory protein of unknown function. In man, the four parathyroid glands
are located just behind the thyroid gland. Ectopic tissue sometimes develops
in the mediastinum or in the neck.
PTH binds to membrane receptors on all target cells. The major effects of PTH are
on three target organs:
1.
Bone: PTH accelerates the removal of Ca2+ and
phosphate from bones (osteolysis by surface osteocytes, resorption of bone by
osteoclasts). PTH stimulates the osteolysis by surface osteocytes causing the
release of Ca2+ for rapid equilibrium with the ECV. After 12 hours,
the delayed effect of PTH, which stimulates the osteoclasts to reabsorb
mineralised bone, sets in.
2.Kidney:
PTH reduces the reabsorption of Ca2+ and phosphate from the proximal tubules, and increases the reabsorption
of Ca2+ from the distal tubules, frequently resulting in an
increased net loss of Ca2+ in the urine. PTH binds to the
basolateral membrane of the tubule cell, and stimulates cAMP, which in turn
diffuses through the cell to the luminal membrane. Here cAMP activates a Ca2+ -reabsorption port.
The glomerular filtration of Ca2+ is easy to calculate, since approximately half the total plasma concentration
is free and filterable (2.5/2 mM). Since 0.94 parts of plasma is water, the
[Ca2+] in the ultrafiltrate is (1.25/0.94) 1.33 mM. A person with
an average glomerular filtration rate of
0.125 l per min will produce a 24-hour ultrafiltrate of 180 litre.
Thus, a total Ca2+ flux of (1.33 × 180 =) 239 mmol or almost 10 000 mg
daily, will pass the glomerular ultrafilter.
Fortunately, almost all Ca2+ is
reabsorbed in the kidney tubules (about 67% is reabsorbed in the proximal, and
the reabsorption of the balance in the distal tubules is regulated by PTH). We
only excrete 100 to 200 mg daily (or 2.5 to 5 mmol daily) in the urine and 50
mg or 1.1 mmol through the skin. This is a daily maximum of 250
mg (or 6 mmol) Ca2+ excretion from the body.
Another drastic action of PTH is on the
proximal tubules, where PTH inhibits phosphate
reabsorption so efficiently that its excretion in the urine increases within 5
minutes.
3.Gut: The PTH action on the gut is indirect. PTH stimulates the renal production of biologically active
vitamin-D (1,25-dihydroxy-vitamin D = calcitriol), which stimulates the active absorption
of Ca2+ and phosphate across the gut mucosa (Fig. 30-3), and
potentiates the action of PTH on bone resorption.
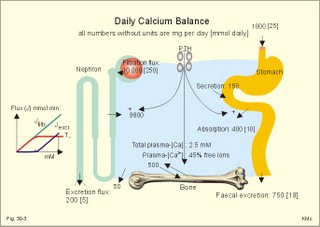
Fig.
30-3: The normal calcium transfer (all numbers are in mg per day or in
[mmol per day]). The balance is 250 mg per day.
The end result of these three actions is an
increase in plasma [Ca2+ ] and a decrease in plasma [phosphate].
Simultaneously, the bone resorption activity is illustrated by a high basic
phosphatase concentration. Cystic areas, corresponding to periostal reabsorption, are visible on bone radiographs
(osteitis fibrosa cystica). Hypercalcaemia predisposes to kidney stones and to metastatic calcification in
synovial membranes and meninges, in the lungs, kidneys, pancreas and
elsewhere. Certain tumours produce PTH-related
protein with sequence homology to PTH. Perhaps this substance is related
to the hypercalcaemia of malignant
processes (see ectopic tumours).
The daily need of phosphate is 18 mmol just
as the daily need of Ca2+ .The human body contains 25 mol of
phosphorous (31 Da) as phosphate. Out of the 25 mol, 20 mol is located in
bones and about 4 mol in muscle and other soft tissues (Fig.
30-4). A daily food intake of 46 mmol of phosphate (equal to the Ca2+ intake) with a secretion of 3 mmol and an intestinal absorption of 39
mmol, leaves (10 + 36) mmol daily for faecal and renal excretion in order to
be in balance. The normal plasma
concentration of phosphate is 1 mM. This implies a daily phosphate filtration
rate (net-flux) of 180 mmol (1 mM*180 l of plasma) - at a glomerular
filtration rate of 0.125 l per min. Phosphate
is a threshold substance with a tubular reabsorption capacity (Tmax)
of 0.1 mmol per min; this means that 80% of the filtered load is reabsorbed.
The maximal daily filtration- reabsorption- and excretion flux is calculated in Fig. 30-4.
Most of the reabsorption of phosphate takes
place in the proximal tubules, where PTH inhibits phosphate reabsorption.
Renal control maintains the phosphate concentration in blood plasma. The Tmax for phosphate is up regulated by high phosphate intake and down regulated by
low phosphate intake in the food. The daily exchange of phosphate from bone is
8 mmol and from soft tissue 16 mmol (Fig. 30-4). – Phosphate depletion may
leads to muscle weakness (cardiac and skeletal muscles) and pathological bone
formation.
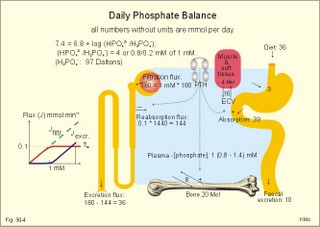
Fig.
30-4: The daily phosphate balance.
The binding of PTH activates adenylcyclase
and this raises the [cAMP], which interacts with protein kinase A. The enzyme
then catalyses the phosphorylation of
effector proteins. PTH is secreted in response to hypocalcaemia, in
particular, a low [Ca2+] (or low [Mg2+]). The major
effects of PTH are to increase plasma [Ca2+] and decrease plasma
[phosphate] through its effect on three target organs: bone, kidney and gut.
– Magnesium Mg2+ is a cofactor in enzyme reactions and important
for the neuromuscular transmission.
3.
Adrenal corticoids
The
adrenal cortex of the adult human has three layers: The outer zona glomerulosa consists of small cells and is narrow, the middle zona fasciculata is wide, and the inner zona reticularis contains a reticulum of interconnected cells. The adrenal
cortex is of mesodermal origin.
Steroids
The
human adrenal cortex produces three types of steroids: Glucocorticoids,
mineralocorticoids, and a minimal amount of sex steroids (androgens and
oestrogens). All steroids are lipid-soluble and easily cross the lipid
membrane. All steroids represent chemical modifications of four-ring
structures (A-D) forming the cyclopentano-perhydro-phenantren.
Steroids bind to specific cytosol-receptor
proteins that are then translocated to the cell nucleus. Here they
reversibly bind to DNA.
The synthesis of glucocorticoids (cortisol and corticosterone) occurs in the zona
fasciculata with a small contribution from zona reticularis. The mineralocorticoid,
aldosterone, is produced in no other region of the cortex than zona
glomerulosa. The synthesis of sex
hormones (androgens and oestrogens) occurs mainly in zona
reticularis. The precursor for these hormones is cholesterol absorbed from the blood HDL and LDL fractions by the cortex cells. Most of the
synthetic reactions involve mixed
oxygenases (belonging to the cytochrome P-450 enzymes) localized in the
endoplasmatic reticulum and in the mitochondria.
During ACTH stimulation the size and number
of cells in the zona fasciculata and zona reticularis increase, mainly because
the cortisol and the sex hormone production increase. The mitochondria,
central ribosomes, vesicular cristae and endoplasmic reticulum grow in these
cells. ACTH activates all steps in corticosteoid hormone synthesis.
A microsomal
desmolase removes C20-21 from the precursors, pregnenolone and progesterone (C21 steroids).
The residues are dehydroepi-androsterone and androstenedione (C19).
These androgens are weak and are converted to a more potent form,
testosterone, in peripheral tissues.
In the zona reticularis, testosterone is
converted to oestradiol (C18), due to removal of a CH3 group by aromatase. The gene for the
human androgen receptors is found on the X chromosome. The receptor
protein has a molecular weight of 98 kDa and is found both in the cytosol and
the nucleus.
CRH & ACTH
The
hypothalamic Corticotropin Releasing Hormone (CRH) stimulates the secretion of
ACTH. Stress stimulates not only the sympatho-adrenergic system with
catecholamine release, but also the CRH/ACTH release with increased secretion
of neuroregulatory peptides and cortisol. This is important, since small
amounts of cortisol have permissive
effects on catecholamines, while inhibiting TSH. Stress also releases growth hormone (GH that stimulates glycogenolysis/glycolysis) and prolactin, both from the acidophilic cells in the adenohypophysis. Prolactin released by
stress is possibly mediated by hypothalamic
histaminergic neurons.
ACTH binds to the cells of zona fasciculata
and activates adenylcyclase, which results in a rise in the cAMP level. The
major effect of ACTH - through increased cAMP level - is to stimulate the
conversion of cholesterol to pregnenolone by desmolase. This is the rate-limiting step in the production of cortical
steroids! Plasma levels of cortisol (hydrocortisone), adrenal androgens and their
precursors rise within three minutes of intravenous ACTH injection. Cortisol
inhibits the release of CRH and ACTH by negative
feedback.
Glucocorticoids
Kendall
isolated cortisone, and Hench & Reichstein directed the first
administration of cortisone and ACTH to patients with rheumatoid arthritis.
The result was a dramatic improvement. They shared the Nobel Prize in 1950.
The serious side effects were recognized later.
The
gene for the human glucocorticoid
receptor is present in chromosome 5.
The receptor protein (94 kDa) is found in both
the cytosol and the nucleus.The effects of a glucocorticoid such as cortisol
are physiologic or pharmacological dependent upon
the dose.
Cortisol is the main endogenous glucocorticoid synthesized in the adrenal cortex.
Cortisol is essential for life and acts
permissively to facilitate the mobilisation of fuels.
Cortisol defends the body against hypoglycaemia evoked by insulin.
Cortisol stimulates hepatic glucose
production (gluconeogenesis).
Cortisol augments the glucagon stimulation
of glycogenolysis.
Cortisol inhibits the glucose uptake in
target cells (GLUT 4 in muscle cells, heart cells and adipocytes).
Cortisol is diabetogenic.
Cortisol is lipolytic and acts permissively
on adrenaline, GH and other lipolytic substances to mobilize triglycerides.
Cortisol also suppresses CRH release and an excess of cortisol may lead to
truncal obesity.
Cortisol induces leptin synthesis in fat
cells. This synthesis limits the appetite by negative feedback.
Cortisol maintains the contractility of striated and cardiac muscle (the Na+-
K+-pump, b-adrenergic
receptors etc). Excess cortisol increases muscle metabolism and reduces muscle mass and strength. Cortisol decreases the ratio of
insulin-sensitive slow oxidative type I muscle
fibres to the fast glycolytis type II-B muscle fibres.
Cortisol reduces the differentiation to
active osteoblasts and the synthesis of collagen in bone matrix and connective
tissue. Cortisol antagonises the action of 1,25-dihydroxy-cholecalciferol and
thus the absorption of Ca2+ from the gut – cortisol excess leads
to osteoporosis.
Cortisol is permissive to the
vasoconstriction of catecholamines and angiotensin II.
Cortisol increases renal bloodflow and GFR.
Cortisol is important for the development of
CNS, skin, gut, bone marrow and lungs. Glucocorticoids stimulate
erythropoiesis.
Cortisol inhibits processes involved in
inflammation, infections, tissue damage, and immune system reactions.
Glucocorticoids inhibit most responses mediated by leucotrienes, NO, PAF,
prostaglandins, and thromboxanes. Therapeutic doses of glucocorticoids are
used to treat more diseases than any other group of drugs, because the
hormones act anti-inflammatory and anti-allergic. The negative effects are
delayed healing of wounds and increased gluconeogenesis with destruction of
tissue proteins.
In plasma, most of the cortisol binds to transcortin, to corticosteroid binding globulin (CBG), or to albumin and only 5% is
free. The protein binding means that cortisol has a long plasma-T½ of more than 70 min. Oestrogens stimulate the production of cortisol binding
proteins. Patients with liver and kidney diseases do not produce enough. –
Transcortin-cortisol complexes activate adenylyl cyclase, and the complex
liberates free colesterol at sites of inflammation.
A low concentration of hormone specific globulin implies a low concentration of the bound hormone fraction, but the free hormone
concentration can still be the same.
Cortisol is converted into
tetrahydrocortisol, cortisone or to 17-ketosteroids. Cortisone is a biologic
analog to cortisol. Exogenous cortisone is an important source of cortisol in
many tissues due to the presence of the enzyme, 11beta-hydroxy-dehydrogenase.
Conjugation with
glucuronic acid or sulphate forms water-soluble products. Only minimal amounts
of the daily cortisol secretion is excreted in the urine. The fraction
excreted in the bile is released to the enterohepatic circuit.Half of the daily cortisol secretion is
excreted in the urine as 17-hydroxycorticoids.
The plasma
cortisol concentration is increased by anxiety, burns, fever, exercise,
hypoglycaemia, pain, severe physical or psychiatric disease, stress and
surgery.The plasma
cortisol concentration decreases promptly following administration of
synthetic glucocorticoids (dexamethasone), which suppress ACTH secretion by
negative feedback.
Mineralocorticoids
Aldosterone is the major mineralocorticoid with corticosterone contributing only little. Aldosterone maintains ECV by
conserving body Na+. When body Na+ is depleted, the fall
in ECV and plasma volume reduces renal bloodflow and pressure. A reduction in
circulating fluid volume recorded in the kidneys releases aldosterone.
Aldosterone promotes the reabsorption of Na+ and increases the secretion of K+ and H+ in the distal
tubules and the cortical
collecting ducts. A rise in plasma-[K+] from normal or a fall in plasma-[Na+] also releases
aldosterone by direct action on zona glomerulosa.
The renin-angiotensin-aldosterone
cascade (Chapter
24) controls the adrenal aldosterone secretion, not the ACTH.
Only a small fraction of the aldosterone
binds to proteins. This makes its half-life short (20 min). Aldosterone is reduced to tetra-hydro-aldosterone in the liver and is conjugated with glucuronic acid.
Aldosterone like all other steroids is
lipophilic, so that it passes easily through the cell membrane. The human
mineralocorticoid receptor is found primarily in the cytoplasm, and its gene
is present on chromosome 4. This
receptor has been cloned revealing a molecular weight of 107 kDa. When
aldosterone is bound to the receptor, the receptor reveals a DNA-binding
site.
The receptor-aldosterone complex
translocates from the cytosol to the nucleus, where it binds to chromatin by
means of the DNA-binding site. This process activates the transcription of the
specific gene producing mRNA, which is then translated into specific proteins.
Following complete transmission, the receptor-aldosterone
complex dissociates from chromatin and each other. The receptor returns to
the cytosol with a hidden DNA-binding
site.
By this process aldosterone promotes the
synthesis of new proteins, that may stimulate the Na+-K+-pump
or facilitate Na+ entry into the tubular cell through integral Na+ -channel proteins in the luminal membrane.
Increased Na+-reabsorption from
the tubules due to aldosterone causes a simultaneous reabsorption of water and
thus an increased ECV, with increased arterial blood pressure. The pressure
rise leads to increased GFR. The rapid filtration flow overrides the high
reabsorptive effect of aldosterone, which down-regulates the size of ECV. –
Beta-adrenergic blockers lower the arterial pressure in part by reducing the
Na+ -retention caused by the renin cascade. Inhibitors of
angiotensin converting enzyme or angiotensin II receptor blockers also lower
the arterial blood pressure. Antagonists of aldosterone at the renal distal
tubule cells directly prevent Na+ -reabsorption and reduce
hypertension.
The ACTH effect is only a moderate
stimulation in acute situations. The action of angiotensin II on aldosterone secretion is much more important.
The adenohypophysis probably produces an aldosterone-stimulating
factor. Dopamine (Prolactin
inhibiting hormone, PIH) is an aldosterone
inhibitor.
Adrenal
sex corticoids are mainly weak adrenal androgens produced
largely in zona reticularis. By conversion to testosterone and
dihydrotestosterone in peripheral tissues, the adrenal androgens play a
physiological role. Also a small oestrogen production is present in healthy
persons. In females, adrenal androgens produce testosterone for normal pubic
and axillary hair development and erythrogenesis.
4.
Adrenal catecholamines
The medulla is a modified
sympathetic ganglion derived from neuroectodermal cells. The development
of the sympathoadrenergic nervous system is stimulated by neural growth
factors.
The adrenal medulla is the source of the
circulating catecholamine, adrenaline. The medulla also secretes small amounts
of nor-adrenaline, normally a neurotransmitter.
The sympathetic
system consists of short preganglionic fibres, which have tropic centers
in the lateral horn of the spinal cord from the first thoracic segment to the
third lumbar segment.
The myelinated nerve fibres form ramus
communicans albus and pass to the paravertebral or lateral sympathetic ganglion chain. Here most of the fibres end on
the postganglionic cell bodies. Some preganglionic fibres pass the sympathetic
chain and reach collateral ganglia such
as the solar plexus, and the ganglion
mesentericus superior and inferior.
In these lateral and collateral relay
stations each preganglionic fibre is in contact with many cell bodies. These
cells have long, postganglionic, unmyelinated fibres serving the different
organs.
The preganglionic fibres to the adrenal
medulla pass all the way to the chromaffin cells in the adrenal medulla. These chromaffin cells (high affinity for
chromium cell stains) are embryological analogues to postganglionic fibres and
the synapse is cholinergic.
Synthesis of catecholamines
Catecholamines are substances consisting of catechol (an
aromatic structure with two hydroxyl groups) linked to an amine. The
catecholamines are synthesises within the chromaffin cell.
The dietary amino acid tyrosine is absorbed as tyrosine from the gut (Fig.
30-5). Tyrosine is also produced in the liver from dietary phenylalanine.
The L-tyrosine is hydroxylated by tyrosine
hydroxylase in the cytosol of the chromaffin cell, and the product is
L-dihydroxy-phenyl-alanine (Dopa, Fig. 30-5). Tyrosine hydroxylase is the
rate-limiting step in the catecholamine production. Dopa is decarboxylated to
dopamine, catalysed by Dopa-decarboxylase. Dopamine is produced in the
cytosol, and then taken up by the chromaffin granules, which contain the
enzyme, dopamine b-hydroxylase.
The enzyme catalyses the formation of nor-adrenaline from dopamine. In most
granules noradrenaline diffuses back into the cytosol, where it is
N-methylated by the enzyme, phenyl-ethanolamine N-methyl-transferase to form
adrenaline. Adrenaline is then stored in the granules as the most important
adrenomedullary hormone. The storage process requires ATP, and catecholamines
are stored with ATP in the granules. - In the newborn the primary end product
in the medulla is nor-adrenaline, but with advancing age there is a dramatic
rise in the adrenaline content. This conversion depends upon cortisol.
The three important catecholamines in humans
are dopamine, epinephrine or adrenaline (Ad), and norepinephrine or noradrenaline (NA in Fig. 30-5).
Dopa is converted to melanin in the melanocytes of the skin, stimulated by melanocytic
stimulating hormone (MSH in Fig. 30-5).
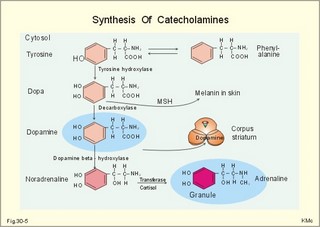
Fig. 30-5: Synthesis of
catecholamines within the chromaffin cell. Tyrosine hydroxylase and dopamine b-hydroxylase
is activated by sympathetic stimulation.
Catecholamines are formed in adrenergic,
noradrenergic and dopaminergic neurons.
The neurons of substantia nigra produce
dopamine, which by axonal transport reaches the striatum (corpus striatum in
Fig. 30-5). Here, dopamine is stored in the granules of the terminals - ready
for liberation in the synapses.
In the sympathetic ganglion cells, dopamine
is just an intermediary step, which is oxidised (dopamine b-hydroxylase)
to form noradrenaline.
Euler identified noradrenaline as the
chemical transmitter from adrenergic nerves, and Axelrod demonstrated the
reuptake of the transmitter after its release from nerve terminals. In 1970
they shared the Nobel Prize with Katz, who explained the role of calcium in
synaptic transmission.
Actions of catecholamines
Catecholamines
act on adrenergic membrane receptors designated a1-, a2-, b1- b2-
and b3-receptors.
The a-receptors
are located on effector cells that are highly sensitive to noradrenaline, less
so to adrenaline, but much less so to isoprenaline (a synthetic
catecholamine). The b-receptors
are located on effector cells that are most sensitive to isoprenaline, but
less so to adrenaline (and noradrenaline). The a1-receptor
acts via calcium and protein kinase C. The a2-receptor
acts through G-protein inhibition of membrane adenylyl cyclase. The b-receptors
are transmembrane glycoproteins, and they use cAMP as second messenger.
Catecholamines prevent hypoglycaemia and restore glucose delivery to the CNS.
Catecholamines increase glucose production by gluconeogenesis in the liver,
and stimulate glycogenolysis in liver and muscle cells. In the absence of
glucagon, adrenaline is important for recovery from hypoglycaemia. Adrenaline
inhibits the insulin-mediated glucose uptake by muscle and fat cells.
Catecholamines stimulate glucagon secretion and inhibit insulin secretion.
Adrenaline activates lipase in fat cells and
thus increases FFA release, b-oxidation
of FFA in muscle and liver and ketogenesis.
Adrenaline increases basal metabolic rate,
nonshivering thermogenesis, and diet-induced thermogenesis during cold
exposure. Catecholamines stimulate proton transfer into the mitochondria and
uncouple ATP synthesis from oxygen utilisation.
Catecholamines increase the heart rate,
contractile force and the cardiac output by stimulation of the adrenergic b1-receptors in the myocardium. Noradrenergic nerve fibres
innervate vessels all over the body, and this system usually has some tonic,
vasoconstrictor activity. The a1-receptors
are located on the surface of vascular smooth muscles. Adrenaline constricts
the arterioles in the cutaneous, renal and splancnic beds.
Catecholamines dilatate the bronchial
airways by stimulation of their adrenergic b2-receptors.
They increase both tidal volume and respiratory frequency. The result is an
increased ventilation with an increased cardiac output.
Catecholamines relax the smooth muscles of the digestive tract (b2-receptors),
but contract the sphincters just
like the sympathetic nerve system.
Finally, adrenaline stimulates the ascending
reticular system (ie, the reticular activating system or RAS) in the brain
stem, keeping us alert and causing arousal
reactions with desynchronisation of the EEG.
Stress responses
Stress comprises severe emotional and physical burdens
(fear, pain, hypoxia, hypothermia, hypoglycaemia, hypotension etc).
The adrenal medulla functions in concert
with the adrenal cortex during stress and during immune system disorders. The
two systems provide useful local cytokines and avoid systemic damages from
accumulated cytokines.
Stress activates the CRH-ACTH-corticoid axis
and adrenergic neurons in the hypothalamus with connections to the sympathetic
nervous system. ACTH is released whereby plasma cortisol is elevated; the
adrenergic stimulus elevates plasma adrenaline. These hormones increase
glucose production.
Noradrenaline and CRH induce a state of
arousal and aggressiveness. CRH inhibits sexual activity and feeding
behaviour.
The sympatho-adrenergic system gives rise to
the fright, flight or fight-reactions,
which are all acute stress
situations. During stress adrenaline induces hyperglycaemia and ketoacidosis
(a diabetogenic hormone). Prolonged stress liberates ACTH via hypothalamic signals. ACTH stimulates the
glucocorticoid secretion through cAMP. Small amounts of glucocorticoids are permissive for the actions of catecholamines.
Acute
stress activates the splancnic nerves and
liberates large amounts of adrenaline from the medulla. Diabetics, who are
developing acute hypoglycaemia, secrete large amounts of catecholamines.
In the endangered individual catecholamines
dilatate bronchioles, inhibit gastrointestinal motility, and dilatate pupils
to improve distant vision.
Exercise
Catecholamines
support the sympathetic system in modifying the circulation during exercise.
During exercise catecholamines promote the use of muscle glycogen, the gluconeogenesis
from lactate, and the mobilisation of FFA as alternative fuel.
During exercise the blood is directed to the
working muscles from the other parts. The resistance vessels of the striated
muscles in hunting predators (and perhaps in humans) are also innervated by
another system. This is the cholinergic,
sympathetic vasodilator system. It is capable of a rapid and appropriate
bloodflow response during hunting. The fall in the a-adrenergic
tone in the muscular arterioles is probably the
most important exercise response in humans.
Metabolism of catecholamines
Plasma
catecholamines are rapidly removed from the
blood and have half-lives in plasma of 20-60 s. This is the combined result of rapid uptake by tissues and inactivation in the liver and vessel walls.
Enzymes inactivate catecholamines by
methylation or by oxidation. Catechol-O-methyl-transferase (COMT) on the surface of the target cells catalyses methylation to
metanephrine, normetanephrine.
Monoamine
oxidase (MAO) in the mitochondria catalyses
oxidative removal of the amino group with formation of vanillylmandelic acid
(VMA) and methoxy-hydroxy-phenyl-glycol (MOPG). The final products in the
urine areVMA and MOPG. The normal excretion of VMA and MOPG in the daily urine
averages 4 and 2 mg.
5.
Vitamin D and bone minerals
Bones
are compared to iron mixed with concrete. The elasticity is due to the collagen (iron) network, and the stiffness (concrete) is due to calcium salts (Ca - hydroxyapatite complex). - Bone
tissue consists of two compartments, bone cells (metabolically active) and the
bone matrix (metabolically inert extracellular compartment). The organic part
of the bone matrix consists of collagen
fibres and ground substance, and
the inorganic part consists of calcium-phosphate-hydroxyapatite.
The atomic weight of calcium is 40, and we
consume 1000 mg (25 mmol) per day. However, we absorb only 400 mg in total.
The net-absorption is only 250
mg, since we secrete 150 mg daily to the intestines. Thus, 750 mg (19
mmol) must be excreted in the faeces every day (Fig 30-3). The net-absorption
is saturable (an active process), since it depends on available Ca2+ -binding protein in the brush border and in the cytosol of the enterocyte.
The synthesis of this protein in the mucosal cells, and thus intestinal Ca2+ -absorption, is induced by active vitamin D and by the parathyroid hormone.
Steroid hormones like vitamin D, exerts their major effects after binding to
specific nuclear receptors and then stimulating the synthesis of mRNA that
codes for cytosolic Ca2+ -binding protein. The basolateral membrane contains two transporters of Ca2+:
A Na+/Ca2+ exchanger, which is more effective at high intracellular [Ca2+],
and a Ca2+-ATPase, which
is the major mechanism at low levels of intracellular [Ca2+]. The
duodenum-jejunum can concentrate Ca2+ against a tenfold
concentration gradient.
The amount of phosphate absorbed and its
concentration in plasma is determined by the amount available in the diet, but
the active transport is somewhat dependent on vitamin D.
High plasma [Ca2+] and
[phosphate] promote bone formation in children. The children increase the
precipitation of Ca-hydroxy-apatite in their bone matrix.
Intracellular
phosphate is an essential component of nucleic
acids, high-energy molecules, cofactors, regulatory phospho-proteins, and
glycolytic intermediates.
The [total calcium] in the blood plasma of
healthy persons is normally 2.5 mM or
100 mg per l. Half the calcium binds to plasma proteins and 5% binds to a
citrate-phosphate-bicarbonate complex. A significant portion (45-50%) is
free ionised calcium ([Ca2+]); this part has critical roles in
neuromuscular function and coagulation. If the plasma [Ca2+] falls
to half its normal level, the body develops increased neural excitability with tetanic cramps (see below). On the other hand, an increase in [Ca2+]
leads to calcification of soft
tissue with heart, kidney and intestinal diseases. The [Ca2+] is
the one variable regulated by parathyroid hormone (PTH) - see Fig.
30-3.
The normal adult contains 27 500 mmol or
1100 g of calcium and 600 g of phosphate. The major part exists in the bones
(1080 g fixed as hydroxyapatite and only 4 g or 100 mmol as exchangeable bone calcium). We exchange this 4 g of calcium five (5)
times a day. The flux between the bones and the ECV (16 l) is approximately
500 mg or 13 mmol daily. This fast exchange regulates [Ca2+] in
plasma. But we also have a small amount of daily bone formation and resorption
(bone remodulation).
In our soft tissue cells 99% of all calcium
is complexed with phosphate in the mitochondria (10-5 mM), or bound to membranes and the endoplasmic reticulum.
The cytosol contains an extraordinarily low [Ca2+]: 10-7 mol per l. The intracellular [Ca2+] is of extreme physiologic
importance as regulator of enzymatic
reactions and secretion, as well as a secondary
messenger for peptide hormones. In general Ca2+ is important to
almost all biological systems: action and membrane potentials, blood clotting,
cell division, cellular secretion, contraction, cytoskeletal rearrangements,
and motility.
Both calcium and phosphate ions are in
equilibrium with calcium-proteinate:
Protein + Ca2+ => 2 H+ + Calcium-proteinate
A hyperventilating patient eliminates
carbonic acid, liberates H+ and reduces [Ca2+] in
plasma, because more Ca2+ binds to protein. Low [Ca2+]
in plasma leads to increased excitability of neuromuscular tissue and to
tetany (tetanic muscle cramps). The treatment is simply to reinhale the
exhaled air (rebreathing) resulting in carbonic acid accumulation (Chapter
17). – As seen from the reaction above, alkalosis reduces and acidosis
increases [Ca2+] in plasma.
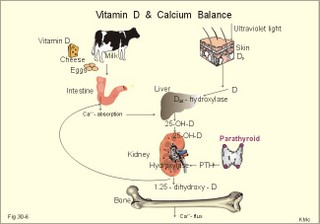
Fig.
30-6: Vitamin D and the Ca2+ balance.
Vitamin D3 (cholecalciferol) from the skin, and vitamin D2 (ergocalciferol)
from plant diet are concentrated in the liver. Both are produced in the skin
by ultraviolet irradiation (Fig. 30-6). In the liver microsomal oxidase simply
hydroxylates the molecules to the weakly active 25-hydroxy-cholecalciferol (25-OH-D). Lack of vitamin D leads to insufficient bone formation, because
the osteoid matrix does not calcify. This disease is called rickets (rachitis) in children and osteomalacia in adults.
This substance is transferred with the blood
to the kidneys, where it is further hydroxylated at the C-1 position to the
most potent form calcitriol (1,25-dihydroxy-
cholecalciferol Fig. 30-6). This is what makes vitamin D a potent steroid
hormone. Vitamin D is stored in muscle and adipose tissue and circulates
in plasma bound to vitamin D-binding
protein.
Vitamin D2 is
derived from vegetable ergosterol and its chemical name is ergocalciferol. Vitamin D3 or cholecalciferol is produced in the skin by ultraviolet irradiation.
The two molecules have equal biological capacity in humans. Vitamin D must be
hydroxylated to the weakly active 25-hydroxy-cholecalciferol in the liver. This substance is transferred with the blood to the liver,
where it is further hydroxylated at the C-1 position to the most potent form 1,
25-dihydroxy-cholecalciferol. This
is exactly what is necessary to make vitamin D a potent steroid
hormone. Vitamin D circulates
in plasma bound to vitamin D-binding
protein and is stored in liver, muscle and adipose tissue.
Vitamin D deficiency causes rickets in children and osteomalacia in adults. The deficiency is caused by insufficient diet, inadequate
absorption as in fat malabsorption, insufficient synthesis in the skin, or
abnormal conversion to its potent form in the liver and kidneys.
Rickets (rachitis) is
extremely rare in the rich part of the world. The epiphyseal plate of the
growing skeleton is inefficiently mineralised and considerably thickened.
Rachitic children have a flat skull with
prominent frontal bones. The sternum protrudes as pigeon
breast or pectus carinatum. The
costal cartilage is enlarged forming the rachitic
rosary. The limbs are shortened and deformed.
Osteomalacia or soft
bones are inadequate mineralisation of the organic bone matrix. Patients
are asymptomatic or they suffer from diffuse pains. Bone X-rays are osteopenic.
High
plasma concentrations of parathyroid hormone, phosphate, and oestrogens
stimulate the renal hydroxylation to active vitamin D. The active vitamin
D-metabolite, the 1,25-dihydroxy-cholecalciferol, stimulates the transport of
Ca2+ across the cell and mitochondrial membranes, and has the
following two effects: 1. Enhanced effect on gut absorption of Ca2+,
so that plasma-[Ca2+] increases, 2. Enhanced effect of PTH on bones. In vitamin D deficiency the low
plasma-[Ca2+] stimulates the parathyroid glands, which leads to secondary
hyper-parathyroidism.
Ca2+ loss continues for months following space
flight. Stimulation of bone deposition requires the stress of muscular
activity as when a person is working against a gravity field. Appropriate
exercise during space missions reduces the total Ca2+ loss.
Puberty growth is essentially stimulated by
the increased production of sex hormones, because the sex steroids stimulate
hypothalamus to secrete more GH. Testosterone and oestradiol are generally
important anabolic hormones.
GH and insulin are also important anabolic
hormones in humans, although GH has many insulin-antagonistic effects. GH is
released as hunger spikes during the
day and during sleep.
Primary hormones for bone remodelling are GH,
PTH, calcitonin and 1,25-D3.
Secondary hormones for bone remodelling are
glucocorticoids, sex hormones, thyroid hormones, prostaglandins (PGE2),
insulin and IGF-I to III (somatomedins).
Bone remodelling is a balance between bone
formation by osteoblasts and bone resorption by osteoclasts and mononuclear
cells. This balance involves the following hormones besides GH:
1. 1,25-Dihydro-cholecalciferol
(1,25-D3) is a D-vitamin, but also a steroid hormone. Kidneys
produce 1,25-D3 when stimulated by PTH. The steroid hormone
increases the calcium absorption from the gut and mobilises Ca2+ from the bones. This increases the [Ca2+] and [phosphate] in
plasma. The 1,25-D3 also increases the renal reabsorption of Ca2+ indirectly.
2. PTH
also increases the plasma [Ca2+]. PTH mobilises Ca2+ from the bones and increases the reabsorption of Ca2+ in the
distal, renal tubule cells while inhibiting phosphate reabsorption in the
proximal tubules of the kidneys.
3. Calcitonin
from the thyroid inhibits bone resorption by blocking the PTH receptors on the
osteoclasts. Thus plasma [Ca2+] and [phosphate] fall.
Cyclic treatment with calcitonin or combined
treatment with 1,25-D3 and PTH improve
bone formation in osteoporosis (ie,
bone atrophia involving both minerals and matrix). Postmenopausal osteoporosis
is treated successfully with calcitonin. Calcitonin is important in bone
remodelling and in treatment of
osteoporosis.
A
parallel to the bone formation by osteoblasts is the dentin formation by
odontoblasts in our teeth. Dentists stimulate dentin formation with Ca(OH)2,
when treating caries.
Pathophysiology
This
paragraph deals with: 1.
Acromegaly and gigantism, 2. Nanism, 3.
Hyperparathyroidism, 4.
Hypoparathyroidism, 5.
Hyperaldosteronism (Conn´s disease), 6.
Cushings disease and syndrome, 7.
Congenital adrenal hyperplasia, 8.
Primary hypoadrenalism, 9.
Secondary hypoadrenalism, 10.
Phaeochromocytoma.
1.
Acromegaly and gigantism
Pituitary
acidophilic adenomas that secrete excess growth hormone (GH) causes gigantism
in children and acromegaly in adults. Rare cases are caused by GHRH excess
release from the hypothalamus. Since pituitary acidophilic adenomas often
contain somatotropic as well as mammotropic cells, the combined adenomas
secrete an excess of both GH and prolactin (causing galactorrhoea in males).
GH excess before the long bone epiphyses
have closed, results in gigantism.
The arms and legs are long, and the patients are tall. Giants may suffer from
deficient sexual development, if the gonadotropic pituitary function also
suffers.
The hypophyseal tumour is demonstrated by CT
scans or by magnetic resonance imaging.
GH stimulates both soft-tissue and skeletal
growth. In adults only bones in the cranium, the face, and the hands and feet
respond the GH excess. Acromegaly often develops around the age of 30 years in
both females and males.
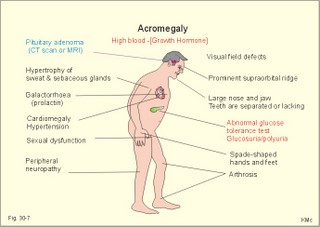
Fig.
30-7: Clinical features of acromegaly.
The calvarium, the maxilla and the mandible
grow (Fig. 30-7). The teeth are separated by spaces and may fall out. The
hands and feet are large and spade-shaped. This is why the adult disorder is called acromegaly (from Greek: extremity-great). Visual field defects are present when the
enlarged hypophysis damages the crossed fibres in chiasma opticum, resulting
in bilateral, heteronymous hemianopsia (Fig. 5-6). The GH concentration in plasma is only increased during stress and as
occasional peak values, but IGF-1 is increased, and impaired
glucose tolerance is diagnostic for a secondary, diabetic condition. There
is often polyuria and glucosuria (Fig. 30-7).
Therapy in cases with growing and
pressure-damaging tumours is trans-sphenoidal or trans-frontal surgery with
post-operative radiotherapy. Medical treatment with octreotide, an analogue to somatostatin (GHIH), is beneficial in
some cases.
2.
Nanism
Nanism
or dwarf growth expresses that the height of an adult is below a certain
limit. In the Anglo-Saxon countries the limit is 1.40 m for females and 1.50 m
for males. Infantile nanism is a term used to indicate that the somatic age of
a child is below its chronological age.
Low pituitary secretion of GH or insensitive
target organ receptor (African pygmies’) result in pituitary dwarfism. Many cases of dwarfism are idiopathic (of
unknown origin). An achondroplastic
dwarf has short limbs and a large head with a flat nose, because of
abnormal growth of cartilage and bone. The abnormal gene is autosomal and
dominant.
3.
Hyperparathyroidism
Primary
parathyroid hyperfunction is almost inevitably due to parathyroid
adenomas or hyperplasia that
secrete an excess of parathyroid hormone,
PTH. Ectopic tumours have been found in the mediastinum and elsewhere.
Excessive secretion of PTH leads to: bone resorption, high [Ca2+]
in plasma, high Ca2+ -excretion in the kidneys with renal stone
formation, bone lesions, and metastatic calcification (Fig.
30-8).
The action of PTH on bone results in
osteitis fibrosa cystica, there is hypercalcaemia with low plasma phosphate, metabolic acidosis and raised PTH levels in
plasma.
Secondary
hyperparathyroidism is an adequate physiological response to
hypocalcaemia by any cause such as renal failure or malabsorption.
Hypercalcaemia has many other causes: hypervitaminosis D, excessive Ca2+ -intake,
leukaemia, myelomata, sarcoidosis and cancer with bone metastases or malignant
tumours producing PTH.
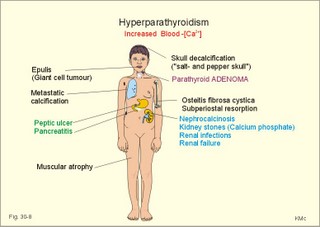
Fig.
30-8: Clinical manifestations of hyperparathyroidism.
4.
Hypoparathyroidism
Hypoparathyroidism is characterised by hypocalcaemia, but most cases of hypocalcaemia are caused by renal failure with phosphate
retention, vitamin D deficiency or resistance to vitamin D, resistance to PTH,
pregnancy and lactation, or calcitonin administration.
Most cases of hypoparathyroidism are iatrogenous (ie, caused by doctors during thyroidectomy or parathyroidectomy).
After seemingly successful surgery there is a dramatic fall in plasma [Ca2+]
and a rise in [phosphate], leading to hypocalcaemia
cramps.
The patient is in tetany with paresthesia,
convulsions, and laryngeal stridor. Without therapy death ensues.
Primary, idiopathic hypoparathyroidism is an
extremely rare autoimmune disease often found in combination with other autoimmune disorders.
Pseudo-hypo-para-thyroidism is used for conditions, where target-organs (bone, kidneys, and gut) are resistant
to PTH. The plasma- [Ca2+ ] is low, plasma-[phosphate] is high, and basic phosphatase activity is high. A PTH
injection does not increase renal phosphate excretion, because the kidneys
are resistant.
Hypoparathyroidism is treated with Ca2+ -infusion and the continuation is a lifelong treatment with PTH
and vitamin D.
5.
Hyperaldosteronism (Conn´s disease)
Patients
with adenoma or hyperplasia of the zona glomerulosa secrete large amounts of
aldosterone - they suffer from primary
hyperaldosteronism. Here, aldosterone works directly on the highly Na+ -loaded distal tubules, so the patient becomes severely K+-depleted. The renal retention of salt and water leads to hypertension.
Loss of K+ and H+ leads to hypokalaemia with muscle weakness and cardiac arrhythmias and to metabolic alkalosis with
hypocalcaemia and tetany.
Plasma aldosterone is elevated although the
patient has a high intake of NaCl. Removal of the neoplasm cures Conn´s
disease.
Secondary
hyperaldosteronism. A patient with serious
congestive heart failure may develop secondary
hyperaldosteronism and become K+-depleted.
At rest the GFR, the renal bloodflow, and the cardiac output are close to
normal. The cardiac patient has an abnormally high Na+ retention
and the accompanying high water retention that increases the ECV. This leads
to oedema and increased venous return to the heart with a small rise in
cardiac output. During exercise the rise in cardiac output is insufficient. The insufficient rise
in bloodflow and blood pressure elicits (via the baroreceptors) a marked
vasoconstriction of the renal circulation. Thus the RBF must decline. Other
vascular beds also constrict markedly during exercise. The key factor to the abnormal
Na+ retention is the reduction
in RBF. The major Na+ reabsorption normally takes place in the
proximal tubules. Therefore the distal tubular fluid contains a small load of
Na+, allowing only a small K+ secretion here. This is in
contrast to the patient with Conn's disease.
6.
Cushings disease and syndrome
Cushing’s
disease is a pituitary disorder with increased
secretion of pituitary ACTH.
Cushing’s
syndrome is
used as a common term for all clinical cases of abnormally high glucocorticoid
concentration, [cortisol],
in the blood plasma.
All clinical cases are divided into two
groups: ACTH-dependent Cushing and non-ACTH-dependent Cushing.
1. ACTH-dependent
Cushing is caused either by pituitary disorder
(Cushing’s disease, see above) or by an ectopic ACTH-producing tumour. This
group of cases is characterized by a high ACTH concentration in the plasma.
2. Non-ACTH-dependent
Cushing. Most clinical cases are caused by
glucocorticoid administration for long periods, but also adrenal tumours
(adenomas and carcinomas) produce excess glucocorticoid. The ACTH secretion is
suppressed.
Clinical
manifestations
The high [cortisol ] in the blood has two major effects on the body:
1. Salt
and water-retention with renal loss of K+ results in a
plethoric tomato face or moon face (Fig. 30-9). The
fluid-retention eventually leads to cardiac
hypertrophy due to prolonged hypertension. There is often peripheral
oedema, if not eliminated by polyuria due to the glucocorticoid-induced diabetes. This type of diabetes is typically
resistant even to large doses of insulin.
2. Catabolism causes muscle wasting, fat accumulation,
osteoporosis with kyphosis, buffalo hump, and fractures. The skin is thin with
ulcers and red stirrer, and there is poor wound healing. There is impaired
fibrocyte formation and capillary resistance.
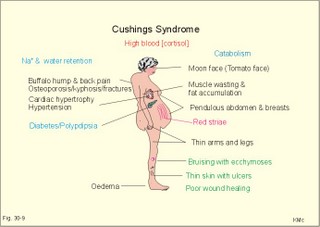
Fig.
30-9: Manifestations of Cushing’s syndrome.
In Cushings
disease there is a pituitary tumour
with enlarged sella turcica or pituitary hyperplasia. The pituitary
corticotrophins produce excessive amounts of ACTH, which leads to bilateral adrenal cortical hyperplasia.
In other cases of Cushing’s syndrome the primary cause is an adrenal tumour or adrenal hyperplasia.
There is a hypersecretion in
cortical zona reticularis and fasciculata.
The patient may suffer from muscular
and bone atrophy due to the catabolic effect of the cortisol surplus. The bone atrophy leads to osteoporosis,
vertebral fractures and necrosis of the hips. There is a delayed
healing of lesions and wounds due to the slow fibrocyte formation. The
skin is thin with purple striae and fragile capillaries. Sodium and water
accumulate in the body, whereas potassium is lost. The nitrogen balance is
negative. The Cushing patient is
often depressed and suffers from insomnia.
The arms and legs are thin and weak due to
the protein catabolic effect, but fat collects as truncal obesity and in the
back and neck regions (bulls neck and buffalo hump). The fluid
accumulation leads to a special look called moon
face or tomato face.
The high blood [cortisol] also increases the
blood [glucose]. A diabetic condition, which
is resistant to even large doses of insulin, develops. The patient suffers
from hypertension and rapidly
develops arteriosclerosis. Female
patients complain of amenorrhoea and poor libido.
The primary therapy is to reduce plasma [cortisol] to approximately 350 nM also as a preparation for surgery. In cases of Cushing’s
disease the tumour is typically removed trans-sphenoidally, and adrenal
adenomas are resected.
The best assessment of increased ACTH production is by measuring the 24-hour
urinary cortisol excretion. Suppression of ACTH and cortisol
is performed with a standard dose of synthetic Glucocorticoid
(dexamethasone), which is potent
enough to suppress the ACTH production, without
influencing the plasma [cortisol].
The principle of the suppression test is to challenge
the hypothalamic-pituitary feedback system. If the system is intact a fall
in blood [cortisol] is expected, and if not (hypothalamic-pituitary Cushing)
the high [cortisol] is maintained.
In healthy persons such a standard dose will suppress ACTH secretion, and lead to
a reduced blood [cortisol]. Such a normal observation is also typical for
patients with a primary adrenocortical
hypertrophy. Dexamethazone does not suppress the high cortisol level of
patients with a damaged feedback system producing
excess ACTH from a pituitary tumour.
Cortisol excess has been treated with
ketoconazole, which inhibits several steps in the corticosteroid synthesis.
Differential
diagnosis:
Alcoholic patients with so-called Pseudo-Cushing look exactly like Cushing’s
syndrome, but the glucocorticoid concentration in the plasma is within
normal limits. The cause is unknown. Cases with plasma [cortisol] in the upper end of the normal scale can be explained in the following way.
Their progressive hepatic failure probably impairs the normal hepatic
destruction of glucocorticoids, whereby the plasma [cortisol] is rising. Such patients should be controlled, because the liver destruction
is likely to proceed, and they become more and more Cushingoid with continued
alcohol abuse.
7.
Congenital adrenal hyperplasia
This
is an autosomal, recessive enzyme deficiency blocking steroid synthetic
pathway of the adrenal cortex. The most frequent of these rare genetic
disorders is the 21-hydroxylase deficiency on chromosome
6. Lack of hydroxylase blocks the conversion of 17-hydroxyprogesterone
into 11- deoxycortisol and further
to cortisol. Instead androstenedione
and testosterone is produced and virilization is an inevitable result. The
impaired cortisol release from the adrenal increases the pituitary ACTH
secretion by negative feedback and more precursor molecules are accumulated
(ie, hydroxyprogesterone, androstenedione and testosterone).
The clinical features are those of an adrenogenital
syndrome. Newborn girls may
show clitoral hyperplasia and labioscrotal fusion, and later some boys develop precocious puberty. Varying degrees
of virilism in females, birdied ladies in circus, or perhaps only hirsutism before puberty occur. The lack of
aldosterone causes salt-and water-loss, which may be life threatening.
A defect in the 11-hydroxylase
enzyme gene also leads to overproduction of androgens and
11-deoxycorticosterone from accumulated precursors. The later has a massive
mineralocorticoid effect.
The therapy is exact replacement of
glucocorticoid activity and any other inefficiency.
8.
Primary hypoadrenalism
Primary
hypoadrenalism is a complete destruction of the adrenal cortex, and thus it
impairs all three lines of steroid production (ie, glucocorticoids,
mineralocorticoids and sex hormones). Primary hypoadrenalism occurs in an
acute and a chronic form.
Acute
hypoadrenalism (ie, adrenal crisis or Waterhouse-Friderichsens syndrome) is a fulminant
infection with shock and massive bleeding all over. The large internal
bleedings are visible at the skin as areas of purpura. The patient dies within
a few hours, if not treated urgently. Hydrocortisone (100 mg) is given intramuscularly. Antibiotics and cortisol is
administered.
Chronic
hypoadrenalism (ie, Addison’s disease) is
hypocorticism due to destruction with atrophy of the entire adrenal cortex by
autoimmune processes, malignancy, infarction or infection. Most cases develop organ-specific
autoantibodies; these cases are associated with many other autoimmune
disorders (eg, diabetes mellitus, hypoparathyroidism, pernicious anaemia,
vitiligo and thyroiditis).
Addison’s
disease is a life-threatening condition with
loss of Na+ and thus also of ECV. Symptoms and signs include:
reduced blood pressure, reduced blood [glucose], tiredness and skin
pigmentation caused by MSH, whose level is increased concurrent with the overproduction of ACTH, due to the decreased negative feedback.
Severe hypotension may develop into cardiovascular collapse, which is called
an Addisonian crisis.
The clinical
manifestations are consequences of the impaired secretion of the three hormone
groups (Fig. 30-10).
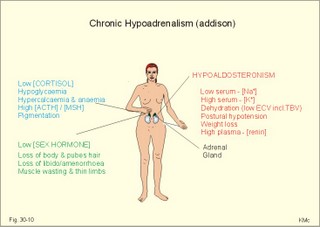
Fig.
30-10: Clinical manifestations of primary, chronic adrenocortical
insufficiency (Addison).
The low cortisol increases the hypothalamic
CRH secretion through feedback and thereby increases ACTH secretion. Fragments
of the ACTH molecule form MSH, which is responsible for the skin
hyperpigmentation. In the absence of glucocorticoids renal phsophate excretion
is depressed.
When
Addison’s disease is suspected, a single plasma ACTH level is often diagnostic, as it will be elevated if
hypocorticism originates in the adrenal
gland (primary) and normal or low in hypothalamic-pituitary
hypocorticism (secondary). - Differentiation between the hypothalamic and
the pituitary hypocorticism can be accomplished by injection of CRH, which stimulates the pituitary directly. - Insulin-induced hypoglycaemia normally recruits the combined
hypothalamic-pituitary axis. Hypoglycaemia is a prolonged stress, which will
normally increase ACTH and glucocorticoid secretion. If there is adrenal gland
insufficiency then this increase in glucocorticoid secretion is not observed. Hypothalamic
and pituitary failure is detected when a dose of CRH restores
glucocorticoid release. - A single
ACTH-injection along with measurement of plasma [cortisol] is a simple
screening procedure for adequate endogenous ACTH secretion. Synthetic ACTH is
injected intravenously. If plasma [cortisol] is normal 30 minutes later, the
disease is not a primary adrenocortical
atrophia. - The metyrapone test is
used when patients are suspected to have Addison’s hypocorticism, but have
reacted normally to the ACTH-test. Metyrapone inhibits the last step in
cortisol synthesis, so 11-dehydrocortisol is accumulated and an acute cortisol
deficiency is created. Plasma-[Cortisol] and -[11-deoxycortisol] is measured two mornings in succession, and the adrenal
11-hydroxylase is inhibited
with 500 mg metyrapone every second hour during 24 hours. The plasma-
[cortisol] must fall while -[11-deoxycortisol] accumulates. The fall in
cortisol biosynthesis increases the CRH and ACTH secretion by negative
feedback, provided the hypothalamic-pituitary system is intact.
Failure to respond to metyrapone suggests a hypothalamic-pituitary defect that
has abolished this feedback.
Substitution
therapy with
gluco- and mineralo-corticoids is necessary for the rest of the patient’s
life.
9.
Secondary hypoadrenalism
This is usually caused by prolonged
corticoid therapy of chronic disorders such as rheumatoid arthritis or COLD. A
rare form of secondary hypoadrenalism is panhypopituitarism.
10.
Phaeochromocytoma
Rarely
some patients have attacks of severe
hypertension., which are caused by a medullary
tumour (a phaeochromocytoma of
chromaffine cells), which liberates large amounts of catecholamines.
Self-Assessment
Multiple Choice
Questions
I.
Each of the following five statements have False/True options:
A. Atropine
blocks muscarinic receptors, and d-tubocurarine blocks nicotinic receptors.
B. b-Receptors are blocked by propranolol.
C. Catecholamines have a half-life in plasma of more than 20 min.
D. The three important catecholamines in humans are adrenaline,
noradrenaline, and DOPA.
E. b1-receptors
are stimulated by adrenaline and located in the myocardium.
II.
Each of the following five answers have False/True options:
ACTH stimulates the liberation of:
A. Hydrocortisone or cortisol
B.
Adrenaline
C.
Aldosterone
D.
Adrenal androgens
E.
Dopamine.
Case
History A
A
man, 49 years of age (height 1.86 m; weight 62 kg) is in hospital due to the
following symptoms and signs. He is nervous and has a diffuse struma. A
characteristic, blowing sound is heard from the thyroid gland with a
stethoscope. The blood pressure is 145/70 mm Hg. An attack of cardiac
arrhythmia is recorded with an ECG. A P-wave frequency above 400 per min is
present during the attack. The concentration of thyroxine in blood serum is
180 nM. The distribution volume for radioactive thyroxine is 8 l. The
elimination rate of this thyroxine is 14% of the total content per 24 hours.
1. Calculate
the serum [thyroxine] in mg
per l.
2. How
much thyroxine is eliminated per 24 hours expressed in mg
daily?
3. Calculate the thyroid plasma clearance of iodide, when the
concentration of free iodide in plasma is 4 mg
per l.
4. Explain the condition of this patient.
Case
History B
A
22-year-old medical student is treated with an intravenous dose of PTH. This
changes his renal excretion flux of two substances and their plasma
concentrations.
1. Describe
the alterations and explain the mechanisms.
2. What
is the diagnosis?
3. Describe
the most likely symptoms and signs of this patient before treatment.
Case
History C
A
female (52 years of age; height 1.68 m; weight 62 kg) is in hospital due to
her third attack of kidney stone pains. The first routine examination with
arterial blood analysis reveals the following. Her blood pH is 7.21 and her
plasma [Ca2+] is 2 mmol per l (mM) in ionised form. Her ionised [Ca2+]
constitute 62% of the total calcium concentration in plasma. The inorganic
phosphate concentration (total) is 0.84 mmol per l of plasma. The patient
excretes 2-3 l of urine per day. pK2 = 6.8 for H3PO4.
1. Calculate
the fractions of primary (H2PO4-) and
secondary (HPO4--) phosphate in her plasma.
Compare the results to the normal mean value of 1 mM
of inorganic phosphate with 20% primary and 80% secondary phosphate at pH =
7.40.
2. Calculate
the total plasma [calcium] of this patient and compare the result to the
normal mean value of 2.5 mM.
3. Why
is the ionised calcium fraction much higher than normal (0.45)?
4. Could
this condition be the result of a classical endocrine disease?
5. Why did this patient develop kidney stones? Was her diuresis normal? If
not explain why.
Try to solve the problems before looking up the answers.
Highlights
· Growth
hormone (GH) produced in the placenta differs from the pituitary GH by a few
amino acid residues. Placental GH suppresses release of maternal, pituitary GH
during pregnancy. Placental GH stimulates maternal metabolism and foetal cell
proliferation and hypertrophia.
· Foetal
thyroid hormones stimulate brain development, and foetal insulin stimulates
foetal growth, cellular glucose uptake and glucose utilisation. Paracrine and
autocrine growth factors are also important for foetal growth: Insulin-like
growth factor-II (IGF-II), nerve growth factors (NGF), epidermal growth factor
(EGF), and platelet derived growth factor (PDGF).
· Growth
hormone and insulin are the most important anabolic hormones in the human
body.
· PTH
binds to membrane receptors on target cells in bone, kidney and gut. The PTH
actions result in hypercalcaemia and hypophosphataemia. The bone resorption is
illustrated by a high basic phosphatase concentration in blood.
· Cortisol
stimulates hepatic glucose production, both glycogenolysis and
gluconeogenesis, lipolysis, formation of FFA and of ketone bodies. Cortisol
inhibits the glucose uptake in target cells (GLUT 4 in muscle cells, heart
cells and adipocytes).
· Therapeutic
doses of glucocorticoids are used for a multitude of diseases such as
inflammations, allergy, malignancy and aplastic anaemia. The negative effects
are delayed healing of wounds and increased gluconeogenesis with destruction
of tissue proteins.
· Aldosterone
is the major mineralocorticoid with corticosterone contributing only little.
Aldosterone promotes the reabsorption of Na+ and increases the
secretion of K+ and H+ in the distal tubular system (ie
the cortical collecting ducts and the connecting segment). A rise in serum - [K+] from normal or a fall in serum- [Na+] releases aldosterone. The renin-angiotensin-aldosterone cascade controls the
adrenal aldosterone secretion, not ACTH.
· Sex
steroids are mainly weak adrenal androgens, which are metabolised to
testosterone and dihydrotestosterone. Also a small oestrogen production is
present in healthy persons.
· Catecholamines
increase the heart rate and the cardiac output by stimulation of the
adrenergic b1-receptors
in the myocardium.
· Noradrenergic nerve fibres innervate vessels all over the body, and this system usually
has some tonic, vasoconstrictor activity. The a1-receptors
are located on the surface of vascular smooth muscles.
· Catecholamines
dilatate the bronchial airways by stimulation of their adrenergic b2-receptors.
Catecholamines increase both tidal volume and respiratory frequency. The
result is an increase in ventilation together with an increase in cardiac
output.
· Catecholamines
relax the smooth muscles of the digestive tract (b2-receptors),
but contract the sphincters just like the sympathetic nerve system.
· Catecholamines
stimulate metabolism (T3). Adrenaline stimulates hepatic
glycogenolysis and lipolysis in adipose tissue. Adrenaline increases the
plasma concentrations of glucose, FFA and ketoacids.
· Adrenaline
stimulates the ascending reticular system (ie, the reticular activating system
or RAS) in the brain stem, keeping us alert and causing arousal reactions with
desynchronisation of the EEG.
· Pituitary
acidophilic adenomas that secrete excess growth hormone (GH) causes gigantism
in children and acromegaly in adults. Rare cases are caused by GHRH excess
release from the hypothalamus. Since pituitary acidophilic adenomas often
contain both somatotropic and mammotropic cells, the combined adenomas secrete
an excess of both GH and prolactin (causing galactorrhoea in males).
· Primary
parathyroid hyperfunction is almost inevitably due to parathyroid adenomas or
hyperplasia that secrete an excess of parathyroid hormone, PTH. Ectopic
tumours have been found in the mediastinum and elsewhere. Excessive secretion
of PTH leads to: bone resorption, high [Ca2+] in plasma, high Ca2+ -excretion in the kidneys with renal stone formation, bone lesions, and
metastatic calcification.
· Chronic
hypoadrenalism (ie, Addison’s disease) is hypocorticism due to destruction
with atrophy of the entire adrenal cortex by autoimmune processes, malignancy,
infarction or infection. Most cases develop organ-specific autoantibodies;
these cases are associated with many other autoimmune disorders (eg diabetes
mellitus, hypoparathyroidism, pernicious anaemia, vitiligo and thyroiditis).
Further Reading
Costanzo,
L S. Regulation of calcium and phosphate homeostasis. Am.
J. Physiol. 275 (Adv. Physiol. Educ.):
S206-S216, 1998.
Änggård,
E. "Nitric oxide: mediator, murderer, and medicine." Lancet 343: 1199-1206, 1994.
Tonegawa,
S. "The molecules of the immune system," Sci.
Am. 253: 122-131, 1985.
Return
to top
Return
to Content
|